Micro-scale biomimicry is revolutionizing material innovation by drawing inspiration from nature’s intricate designs.
This approach is helping startups and innovators create unique products that stand out in competitive markets.
Understanding Micro-Scale Biomimicry
Key Points
- Micro-scale biomimicry involves mimicking nature’s designs at a microscopic level.
- It leads to the development of materials with enhanced properties.
- Applications range from biomedicine to aerospace.
- Advanced techniques like micro-computed tomography (microCT) are crucial.
- Startups are leveraging this approach for innovative solutions.
Definition and Importance
Micro-scale biomimicry refers to the practice of emulating natural structures and processes at a microscopic level to develop new materials and technologies. This approach is significant because it allows for the creation of materials with properties that are difficult to achieve through traditional methods. By studying and replicating the intricate designs found in nature, researchers can develop materials that are stronger, more flexible, and more durable.
One of the key benefits of micro-scale biomimicry is its potential to solve complex engineering problems. For example, materials inspired by the structure of spider silk or the surface of lotus leaves can exhibit remarkable strength and water-repellent properties. These innovations can lead to advancements in various fields, including biomedicine, aerospace, and environmental engineering.
Moreover, micro-scale biomimicry promotes sustainability by encouraging the use of natural designs that are inherently efficient and environmentally friendly. This approach aligns with the growing demand for sustainable and eco-friendly products, making it an attractive option for startups and innovators looking to make a positive impact.
Techniques and Tools
Several advanced techniques and tools are essential for micro-scale biomimicry. One of the most important is micro-computed tomography (microCT), which allows researchers to visualize and analyze natural structures in three dimensions. This non-destructive imaging technique provides detailed insights into the internal and external features of materials, enabling precise replication of natural designs.
Another crucial tool is high-resolution 3D lithography, which is used to create micro-architected materials with intricate designs. This technique allows for the fabrication of materials with complex geometries and fine details, making it possible to replicate the intricate structures found in nature. By combining microCT and 3D lithography, researchers can develop materials with enhanced properties, such as increased strength, flexibility, and durability.
Additionally, computational modeling and simulation play a vital role in micro-scale biomimicry. These tools enable researchers to predict the behavior of materials under different conditions and optimize their designs for specific applications. By leveraging these advanced techniques and tools, innovators can push the boundaries of material science and develop groundbreaking solutions inspired by nature.
Applications and Impact
The applications of micro-scale biomimicry are vast and diverse, with significant potential to impact various industries. In biomedicine, for example, materials inspired by natural structures can be used to create biocompatible implants, drug delivery systems, and tissue engineering scaffolds. These innovations can improve patient outcomes and advance the field of regenerative medicine.
In the aerospace industry, micro-scale biomimicry can lead to the development of lightweight, high-strength materials that enhance the performance and safety of aircraft and spacecraft. By mimicking the structure of bird bones or insect wings, researchers can create materials that are both strong and lightweight, reducing fuel consumption and increasing efficiency.
Environmental engineering is another area where micro-scale biomimicry can make a significant impact. Materials inspired by natural surfaces, such as the self-cleaning properties of lotus leaves, can be used to develop water-repellent coatings and filtration systems. These innovations can help address pressing environmental challenges, such as water scarcity and pollution, by providing sustainable and efficient solutions.
Challenges in Product Development
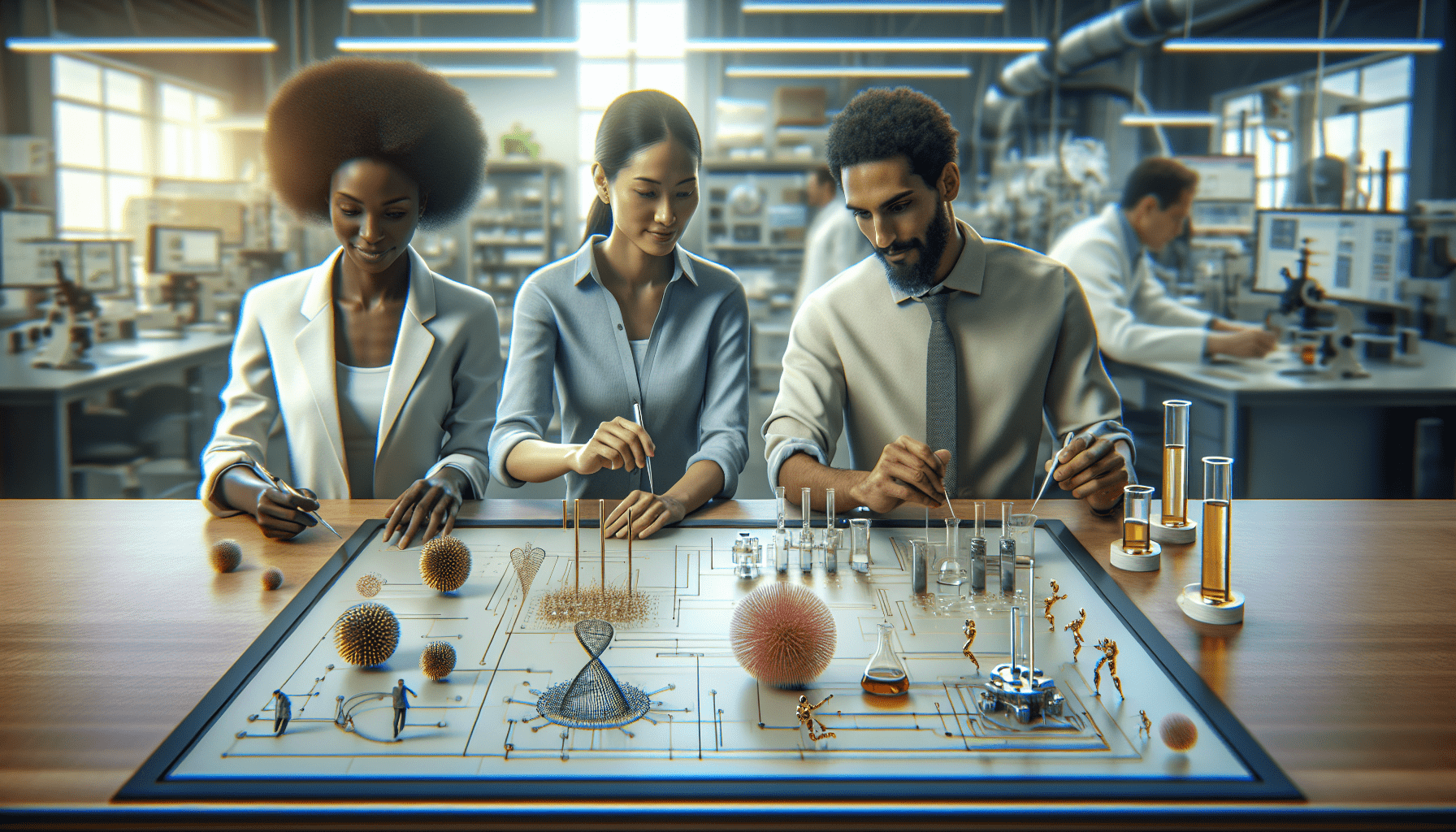
Complexity of Natural Designs
One of the most challenging aspects of product development in the context of micro-scale biomimicry is the complexity of natural designs. Nature’s structures are often intricate and multifaceted, making them difficult to replicate accurately. This complexity can pose significant challenges for researchers and engineers attempting to develop materials with similar properties.
For example, the structure of a spider’s silk is composed of multiple layers and intricate patterns that contribute to its remarkable strength and flexibility. Replicating this design at a microscopic level requires advanced techniques and precise control over the fabrication process. The complexity of natural designs can also lead to increased costs and longer development times, making it challenging for startups and small companies to compete in the market.
Material Limitations
Another significant challenge in micro-scale biomimicry is the limitations of available materials. While nature provides a wealth of inspiration, not all natural designs can be easily replicated using existing materials. Some natural structures rely on unique properties that are difficult to achieve with synthetic materials, limiting the potential applications of micro-scale biomimicry.
For instance, the adhesive properties of gecko feet are due to the presence of microscopic hair-like structures called setae. Replicating these structures using synthetic materials has proven to be challenging, as it requires materials with specific mechanical and chemical properties. Overcoming these material limitations is crucial for the successful implementation of micro-scale biomimicry in product development.
Scalability and Manufacturing
Scalability and manufacturing are also significant challenges in the field of micro-scale biomimicry. Developing materials with intricate designs at a microscopic level often requires specialized equipment and techniques, which can be costly and time-consuming. Scaling up the production of these materials to meet commercial demands can be a daunting task for startups and small companies.
Moreover, the manufacturing processes for micro-scale biomimicry often involve multiple steps and precise control over various parameters. Ensuring consistency and quality in the final product can be challenging, especially when dealing with complex designs. Addressing these scalability and manufacturing challenges is essential for the widespread adoption of micro-scale biomimicry in the industry.
Steps to Implement Micro-Scale Biomimicry
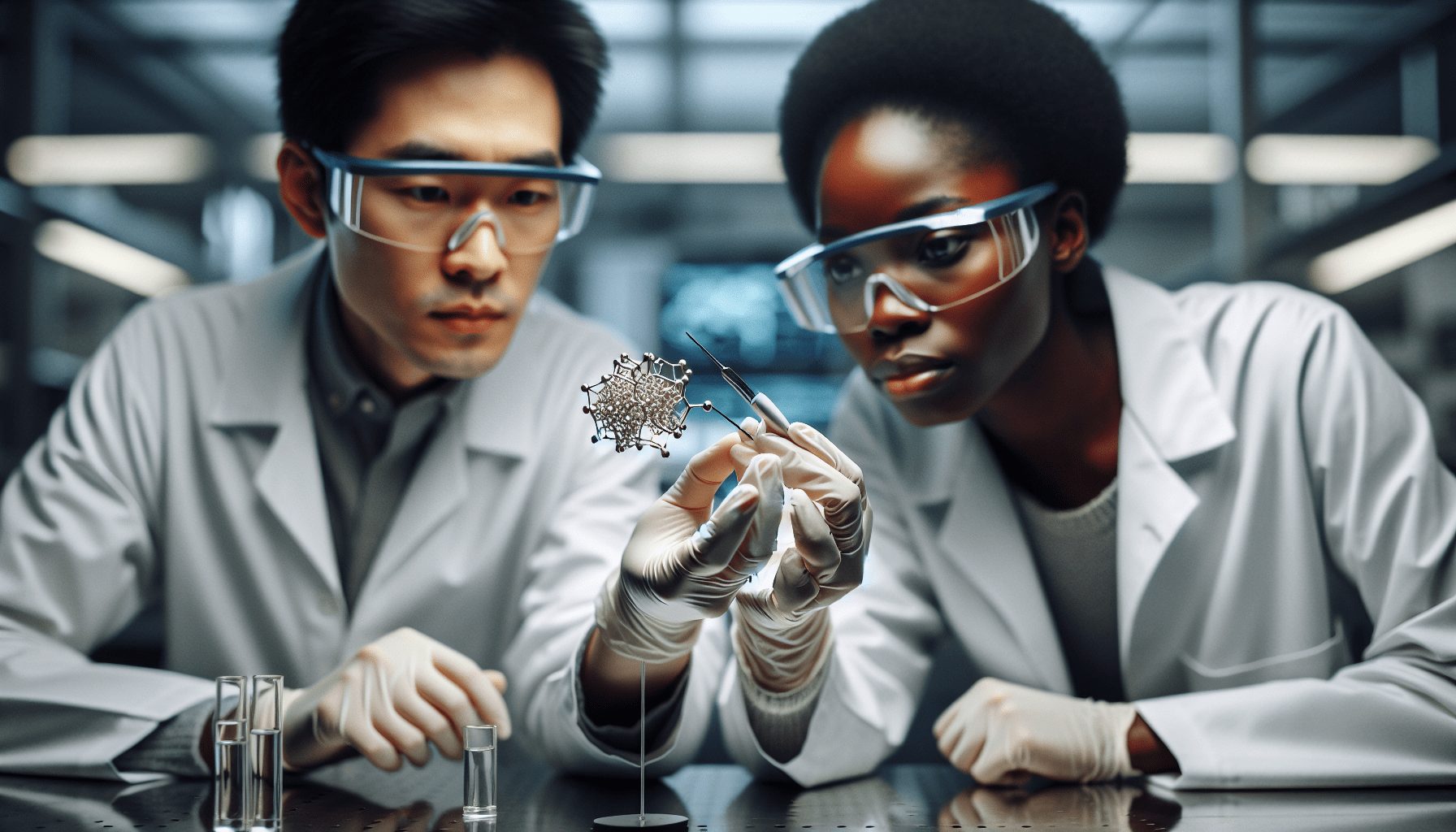
Step 1: Research and Analysis
The first step in implementing micro-scale biomimicry is conducting thorough research and analysis of natural structures. This involves studying the intricate designs found in nature and understanding their underlying principles. Researchers can use advanced imaging techniques, such as microCT, to visualize and analyze these structures in three dimensions.
By gaining a deep understanding of natural designs, researchers can identify key features and properties that can be replicated in synthetic materials. This research phase is crucial for developing a solid foundation for the subsequent steps in the implementation process. It also helps in identifying potential challenges and limitations that need to be addressed.
Step 2: Design and Fabrication
Once the research and analysis phase is complete, the next step is designing and fabricating the micro-architected materials. This involves using advanced techniques, such as high-resolution 3D lithography, to create materials with intricate designs at a microscopic level. Computational modeling and simulation can also be used to optimize the designs for specific applications.
During the fabrication process, it is essential to ensure precise control over various parameters to achieve the desired properties. This may involve multiple iterations and adjustments to fine-tune the design and fabrication process. Collaboration with experts in material science and engineering can be beneficial in overcoming technical challenges and achieving the desired outcomes.
Step 3: Testing and Optimization
The final step in implementing micro-scale biomimicry is testing and optimizing the fabricated materials. This involves conducting rigorous testing to evaluate the performance and properties of the materials under different conditions. Mechanical testing, such as tensile and compression tests, can provide valuable insights into the strength, flexibility, and durability of the materials.
Based on the test results, researchers can make necessary adjustments and optimizations to improve the performance of the materials. This iterative process of testing and optimization is crucial for ensuring the final product meets the desired specifications and requirements. It also helps in identifying any potential issues or limitations that need to be addressed before commercial production.
FAQs
What is micro-scale biomimicry?
Micro-scale biomimicry involves emulating natural structures and processes at a microscopic level to develop new materials and technologies. It draws inspiration from nature’s intricate designs to create materials with enhanced properties.
What are the benefits of micro-scale biomimicry?
Micro-scale biomimicry offers several benefits, including the development of materials with improved strength, flexibility, and durability. It also promotes sustainability by encouraging the use of efficient and eco-friendly designs found in nature.
What are the challenges in implementing micro-scale biomimicry?
Challenges in implementing micro-scale biomimicry include the complexity of natural designs, material limitations, and scalability and manufacturing issues. Overcoming these challenges requires advanced techniques and precise control over the fabrication process.
How is micro-computed tomography (microCT) used in micro-scale biomimicry?
Micro-computed tomography (microCT) is used to visualize and analyze natural structures in three dimensions. This non-destructive imaging technique provides detailed insights into the internal and external features of materials, enabling precise replication of natural designs.
Future of Micro-Scale Biomimicry
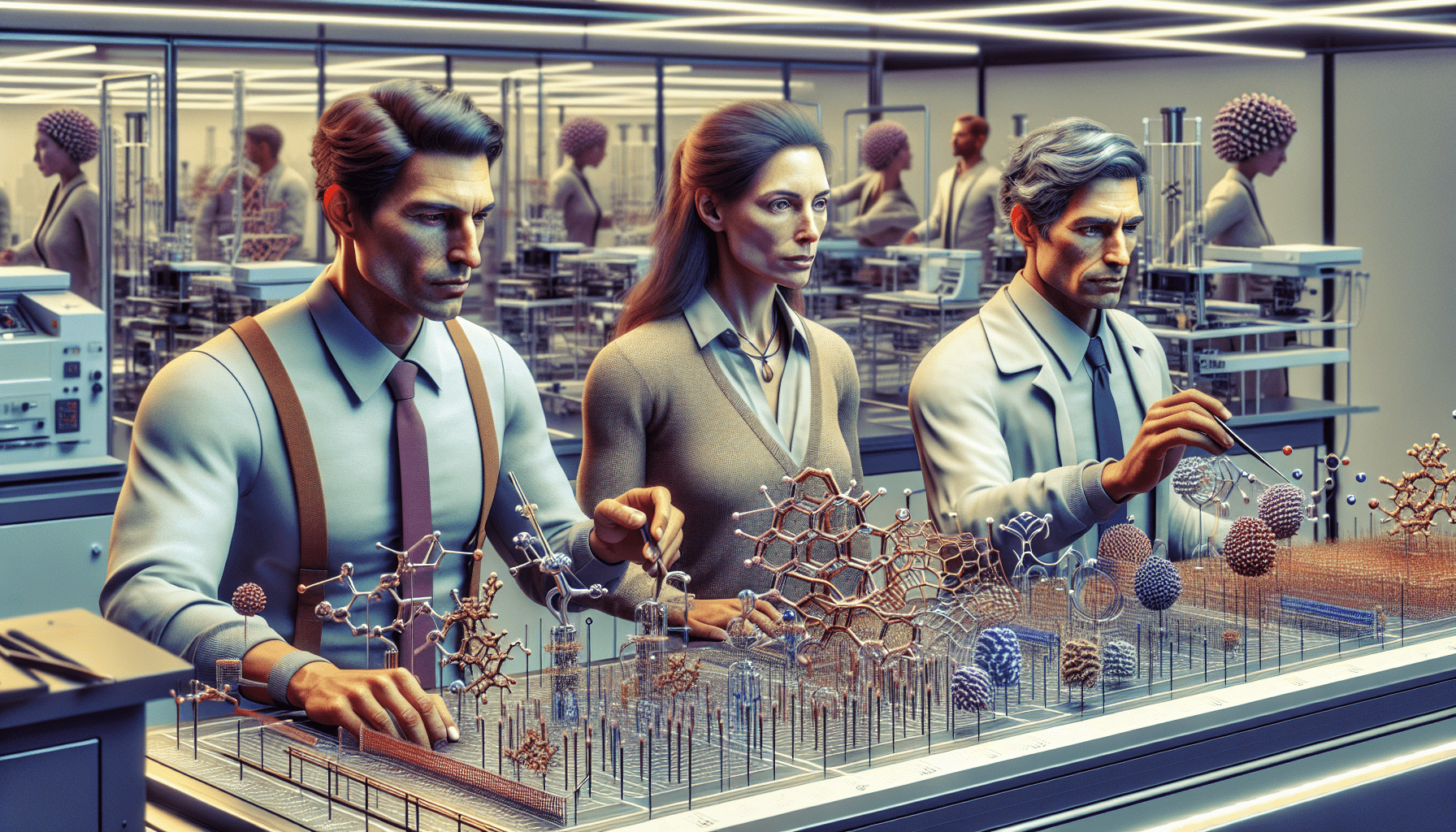
The future of micro-scale biomimicry holds exciting possibilities, driven by advancements in technology and a growing focus on sustainability. Here are five robust predictions for the future:
- Increased Adoption in Biomedicine: Micro-scale biomimicry will lead to the development of advanced biocompatible materials for implants, drug delivery systems, and tissue engineering.
- Advancements in Aerospace Materials: Lightweight, high-strength materials inspired by nature will enhance the performance and safety of aircraft and spacecraft.
- Sustainable Environmental Solutions: Nature-inspired materials will address environmental challenges, such as water scarcity and pollution, through innovative filtration and water-repellent technologies.
- Integration with Additive Manufacturing: The combination of micro-scale biomimicry and additive manufacturing will enable the production of complex, customized materials with enhanced properties.
- Expansion into New Industries: Micro-scale biomimicry will find applications in new industries, such as consumer electronics and energy, driving innovation and sustainability.
More Information
- Knots Smaller Than Human Hair Make Materials Unusually Tough – An article on the development of tough materials using micro-scale knots.
- The Garage of Small Things; nanotechnology, biomimicry and design practice – An exploration of advanced material research and biomimicry.
- Looking deep into nature: A review of micro-computed tomography in biomimicry – PubMed – A review of the use of microCT in biomimicry research.
Disclaimer
This is an AI-generated article with educative purposes and doesn’t intend to give advice or recommend its implementation. The goal is to inspire readers to research and delve deeper into the topics covered in the article.
- Burnout in Remote Teams: How It’s Draining Your Profits - January 27, 2025
- Signs You’re Understaffed - January 20, 2025
- The Cost of Silence: Communicating Negative Feedback - January 13, 2025